Spin relaxation phenomena
Spintronics i.e. the use electron spin for information processing is motivated by the orders of magnitude longer conservation of electron spin alignment in metals or semiconductors as compared to their momentum conservation time. The survival of spin orientation is characterized by the spin relaxation times. Our research group studies these material dependent characteristic times also experimentally and theoretically with collaborators.
Electron spin resonance (ESR) spectroscopy:
To measure electron spin relaxation time two conventional methods are generally used: the nonlocal Hanle spin precession method, which requires device fabrication, ferromagnetic leads and can be applied mainly on low dimensional materials or thin films. On the other hand for bulk samples electron spin resonance is being used, which does not require special treatment of the materials, thus air sensitive samples without extra “tricks” can be examined too. ESR measurements are carried out usually in moderate magnetic fields (~0.3 T for X-band), where the transition between the Zeeman levels can be accessed with microwave photons. During an ESR experiment the electron spin relaxation time, the g-factor and the static spin susceptibility can be measured as a function of temperature. With the extension of the method, it is possible to carry out measurements in lower (typically L and S-bands) and higher (Q, W-bands, 220 GHz, etc.) magnetic fields and thus the frequency dependence of the above mentioned quantities can also be examined. The method is not limited to the detection of paramagnetic centers (EPR), but also applicable to study conducting electrons (CESR). We used the latter method to observe the ultralong spin relaxation time in graphite, to examine the relaxation anisotropy in graphite and its intercalated derivatives.
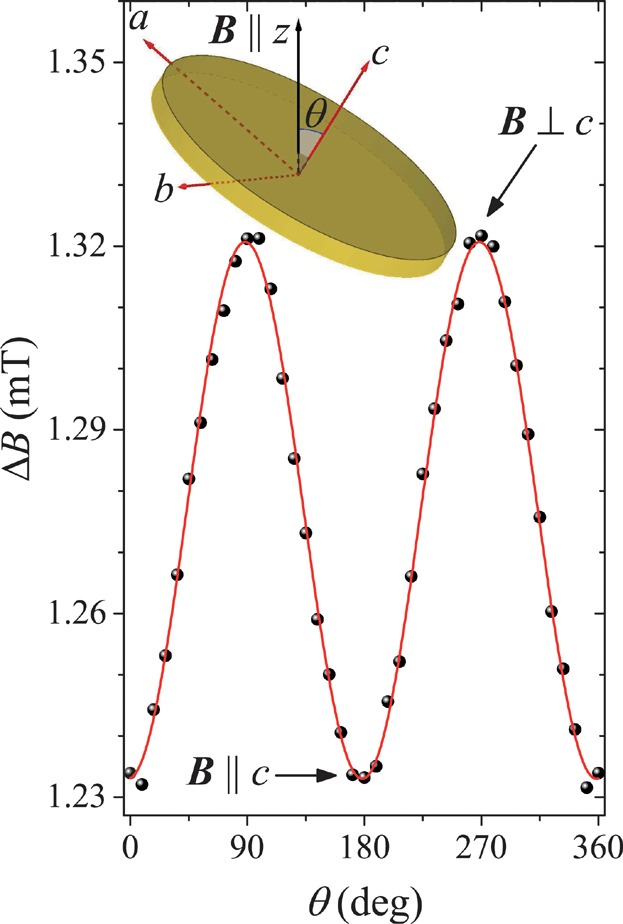
Doped few layer graphene and single walled carbon nantotube samples were also probed by this method, where the elevation of the Fermi sea and its consequences were studied. Furthermore, the method is also capable of detection of free radicals, to manipulate embedded quantum systems (e.g. NV centers in diamond) and much more.
The unified theory of spin relaxation:
Spin relaxation is conventionally discussed using two different approaches for materials with and without inversion symmetry. In materials with inversion symmetry, we can describe the spin relaxation mechanism via Elliot—Yafet theory which predicts the spin relaxation time is proportional to the momentum relaxation time. However, without inversion symmetry, D’yakonov and Perel’ description can be used, and in this case the inverse of the spin relaxation time is proportional to the momentum relaxation time. Our group has shown that formally an analogy between these two different descriptions can be found. We have combined Monte Carlo method and diagrammatic calculation based approaches in our study.
References
Markus, BG; Szolnoki, L; Ivan, D; Dora, B; Szirmai, P; Nafradi, B; Forro, L; Simon, F: Anisotropic Elliott-Yafet theory and application to KC8 potassium intercalated graphite, PHYSICA STATUS SOLIDI B-BASIC RESEARCH 253: 12 pp. 2505-2508., 4 p. (2016)
Szirmai, P; Fabian, G; Dora, B; Koltai, J; Zolyomi, V; Kurti, J; Nemes, NM; Forro, L; Simon, F: Density of states deduced from ESR measurements on low-dimensional nanostructures; benchmarks to identify the ESR signals of graphene and SWCNTs, PHYSICA STATUS SOLIDI B-BASIC RESEARCH 248: 11 pp. 2688-2691., 4 p. (2011)
Szolnoki, L; Dora, B; Kiss, A; Fabian, J; Simon, F: Intuitive approach to the unified theory of spin relaxation, PHYSICAL REVIEW B 96: 24 Paper: 245123, 9 p. (2017)
Szolnoki, L; Kiss, A; Dora, B; Simon, F: Spin-relaxation time in materials with broken inversion symmetry and large spin-orbit coupling., SCIENTIFIC REPORTS 7: 1 Paper: 9949, 10 p. (2017)
Advanced magneto-optical spectroscopy
Photoluminescence (PL) spectroscopy
Photoluminescence is the process of absorption of a photon followed by the emission of a photon. The emitted photon has lower energy, thus higher wavelength, than the absorbed one. By analyzing the absorption and emission wavelength of the photoluminescence one can make assumptions about the materials the sample contains. The method can also be used to characterize the optical and electronic properties of semiconductors and molecules. To conduct PL measurements we use a cw pump laser set up to pump a DYE laser and a Ti:Sapph laser. With the tunable lasers, we can excite the materials in almost the whole visible range and part of NIR, we can detect the PL signal in the whole visible and NIR range. With the aid of this system, we examine the PL signal of carbon nanotubes in different environments, conduct measurements routinely at 77K temperature and, combined with a magnetic field and microwave, we are able to make ODMR measurements.
The microwave photoconductivity decay
The microwave photoconductivity decay is an easy and accurate way to examine the charge carrier lifetime and the number of charge carriers generated in a semiconductor. By putting the sample in a microwave field and measuring the reflected microwave during illumination of the sample with a pulsed laser the calculation of the carrier lifetime is done by analyzing the decay of the signal. This method is widely used by the industry, mainly in the quality measurement of silicon wafers. With the aid of this setup, we conduct measurements using semiconductor materials such as methyl ammonium led halides, which is a candidate material for the solar cells of the future.
Nitrogen vacancy centers
Nitrogen vacancy (NV) centers are complex point defects in diamond lattice built up by a vacancy and a neighboring nitrogen atom. These artificial atom like systems can occur in natural diamond but can be produced directly. In our lab, we create such samples and investigate their magneto-optical properties using photoluminescence (PL), electron spin resonance (ESR), and optically detected magnetic resonance (ODMR) techniques. Our goal is to create diamonds with a high concentration of NV centers for later ensemble quantum technologic applications. Promising applications (with a lot of unanswered questions) of such systems are high sensitivity magnetic and electric field sensing, temperature and stress sensing based on the sharp resonance in the ODMR spectrum. Their absorption and photoluminescence in the visible and near IR range makes them suitable for quantum information processing applications. Carrying out ESR and ODMR measurements the spin relaxation time of the NV centers can be determined, which has a great impact on the potential applications. Combining the high power microwave fields, the accurately controlled magnetic field and optical excitation covering the whole absorption spectrum we are able to carry out a detailed analysis of NV centers even at low temperatures using nitrogen-cooled coplanar waveguides as microwave irradiation circuit.
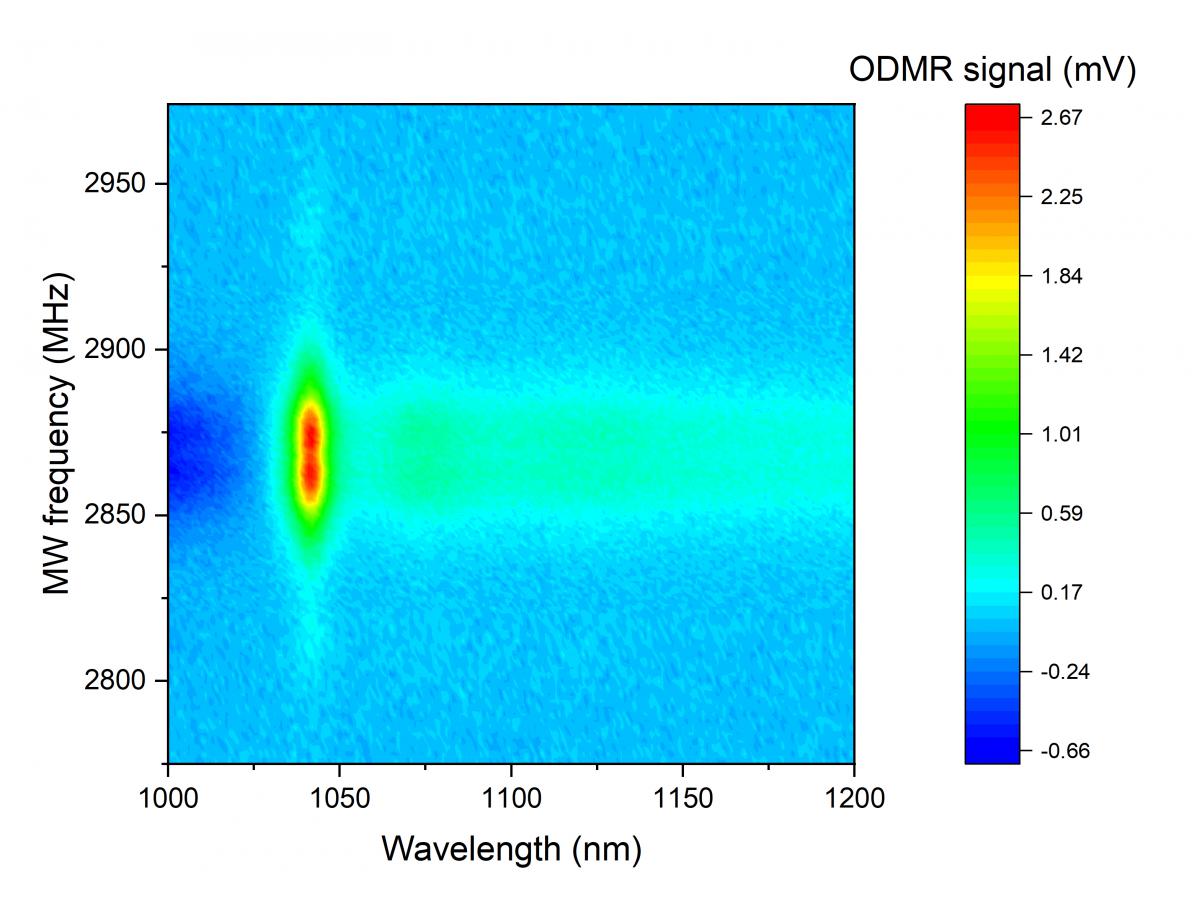
Thermotherapy
Nanomagnetic hyperthermia (NMH) emerged as a potential tool for tumour treatment in cancer therapy. It involves a targeted delivery of ferrite nanoparticles to the affected tissues and its heating with an external alternating magnetic field which warms selectively and efficiently the embedding tissue only. The success of NMH relies heavily on several key medical factors such as the affinity of the tumour tissue to overtemperature and how specifically the ferrite is delivered to the desired location. On the physics side, the method depends on the accurate control and knowledge of the power that the ferrite dissipates. To obtain this information, most methods involve modeling of the exciting RF magnetic field and this information is combined with the knowledge of the magnetic properties of the delivered ferrite or the delivered heat is determined from calorimetry, which however is an invasive and inaccurate method. Therefore, we developed a brand-new method that we can obtain the absorbed power directly. It is based on reflectometry i.e. we determinate the absorbed power from the resonator’s quality factor change caused by the nanoparticles. In order to obtain the required quality factors, we use short (100µs) radiofrequency (RF) pulses, and measure the downconverted transient coming from the resonator. This method is more accurate than the frequency sweeping, thus there are several applications finding the exact location of a buried ferrite sample.
